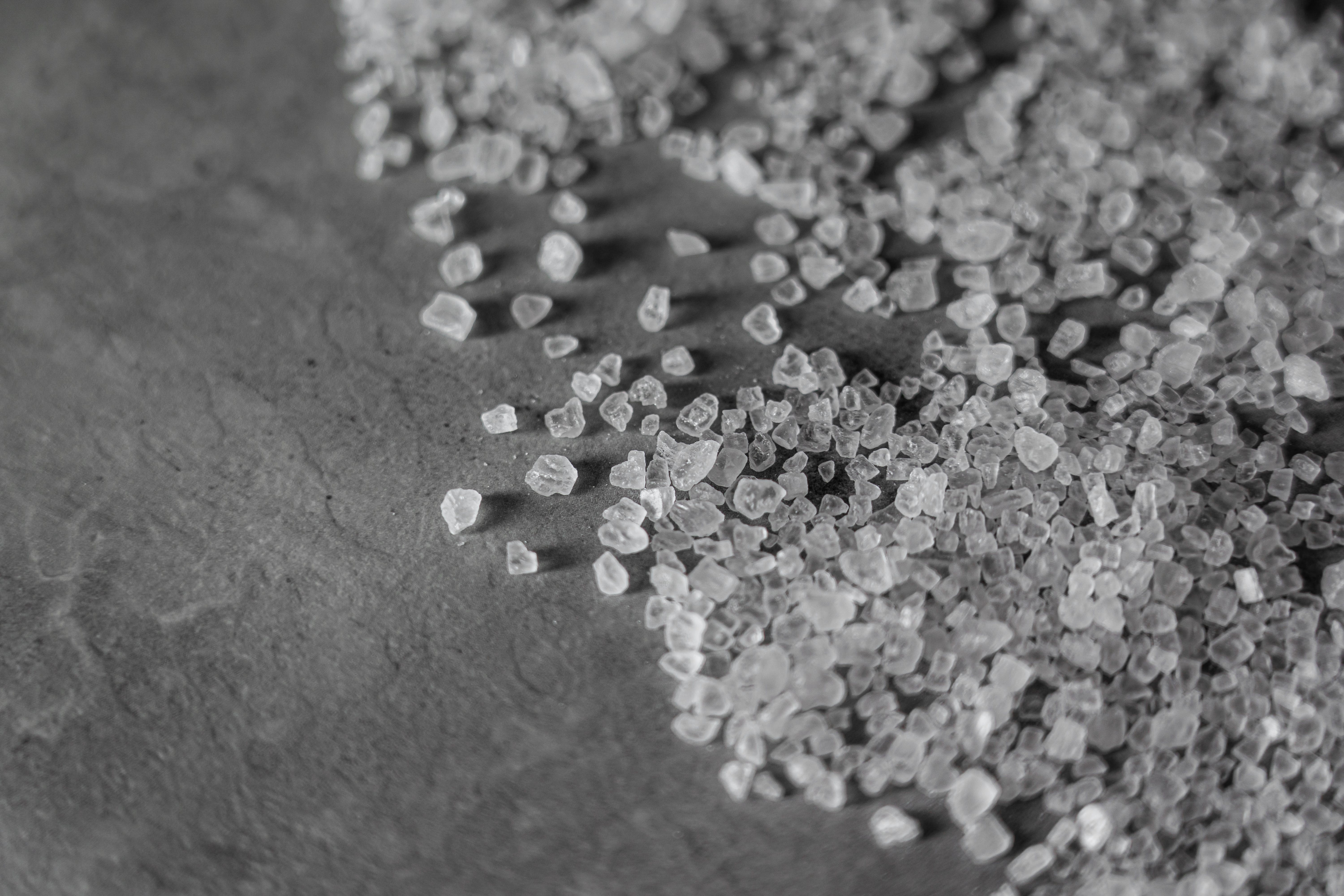
Researchers at the City University of Hong Kong found the secret to a more efficient, less expensive approach to keeping massive computer systems cool: Just add salt.
A growing concern in computing is the enormous amount of heat generated in ever-increasing global computer arrays churning out more than 2 zettabytes per week.
Such gigantic processing capacity leaves an enormous carbon footprint. Commercial cloud providers utilize cooling systems requiring billions of gallons a year to maintain optimal temperatures.
According to the MIT Press Reader, cooling processes account for more than 40% of electricity usage. A single data center can consume the same amount of electricity as 50,000 homes.
“As a result, the cloud now has a greater carbon footprint than the airline industry,” anthropologist Steven Gonzalez Monserrate wrote in an MIT Press article last year.
Wei Wu, a professor in the School of Energy and Environment at the Hong Kong university, devised a system with several colleagues that improves upon current heat dissipation technology.
In a paper published Oct. 31 in the journal Device, Wu described a process in which water containing the salt lithium bromide relies on evaporation and reabsorption to keep temperatures low.
The process is “a passive thermal management strategy that relies on moisture desorption from hygroscopic salt solutions through a protective membrane that only allows water vapor to pass through,” Wu said.
The porous membrane is set in a structure that prevents any salty solution from contact with computer components. A heat sink directs accumulated heat away from sensitive electronics.
Wu, in his paper, “Membrane-encapsulated, moisture-desorptive passive cooling for high-performance, ultra-low-cost, and long-duration electronics thermal management,” said the device “can spontaneously and quickly recover its cooling capacity by absorbing water vapor from the air during off hours.” He compared the process to nature, where mammals regulate body temperature by sweating and rehydrating.
The high moisture absorption capacity and low cost of the lithium bromide-treated water offers a clear advantage over competing cooling strategies using hydrogels and metal-organic frameworks. Those systems require time-consuming regeneration rates and require active replenishment of water supplies.
“In contrast, hygroscopic inorganic salts, such as LiCl and LiBr, show promise in addressing these issues due to their extremely high moisture absorption capacity and low cost,” Wu said.
Applying the process to a single computer, the researchers measured an improvement of more than 32% in performance. They also said the process was completed “with a record-high cost effectiveness.”
“Poor thermal management can cause tremendous heat accumulation within electronic devices, resulting in the loss of functionality and, eventually, device failure,” Wu said. “We demonstrate that the proposed strategy is capable of offering a long-duration stable cooling capacity without solution leakage and corrosion, which can suppress the temperature rise of an emulated heater with a record-high cost effectiveness compared with state-of-the-art passive cooling strategies.”
The cooling strategy, Wu said, is cost effective and highly scalable, and “can be useful for various cooling applications with few technological barriers.”
Source Tech Xplore